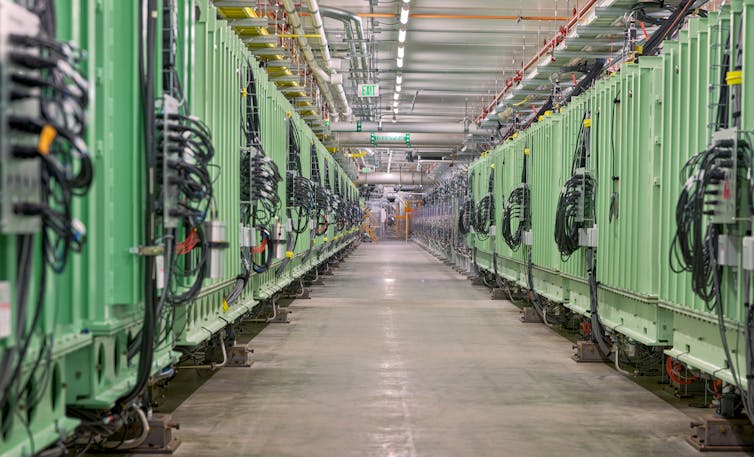
Facility for Rare Isotope Beams
Artemis Spyrou, Michigan State University and Sean Liddick, Michigan State University
The word magic is not often used in the context of science. But in the early 1930s, scientists discovered that some atomic nuclei – the center part of atoms, which make up all matter – were more stable than others. These nuclei had specific numbers of protons or neutrons, or magic numbers, as physicist Eugene Wigner called them.
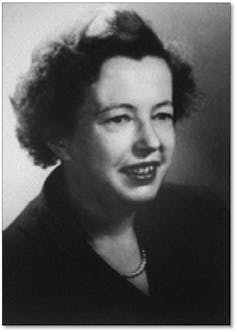
Argonne National Laboratory, CC BY-NC-SA
The race to figure out what made these nuclei so stable began. Understanding these magic numbers would allow scientists to predict the properties of other nuclei, such as their mass or how long they are expected to live. With that, scientists could also predict which combinations of protons and neutrons can result in a nucleus.
The solution to the puzzle came in 1949 from two directions simultaneously. In the U.S., physicist Maria Goeppert Mayer published an explanation, at the same time as a group of scientists led by J. Hans D. Jensen in Germany found the same solution.
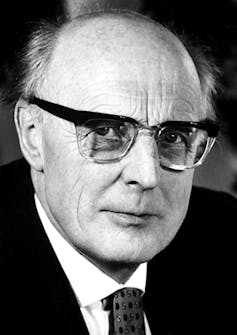
The Nobel Foundation
For their discovery, the two physicists each got a quarter of the 1963 Nobel Prize in physics. We’re two nuclear scientists whose work is built on Goeppert Mayer’s and Jensen’s discoveries 75 years ago. These magic numbers continue to play an important role in our research, only now we can study them in nuclei that live for just a fraction of a second.
Stability in the atom
The atom is a complex system of particles. It’s made up of a central nucleus consisting of protons and neutrons, called nucleons, with electrons orbiting around the nucleus.
Nobel prize-winning physicist Niels Bohr described these electrons in the atom as existing in a shell structure. The electrons circulate around the nucleus in particular energy levels, or orbits. These orbits have specific energies, and each orbit can hold only so many electrons.
Chemical reactions result from interactions between the electrons in two atoms. In Bohr’s model, if an electron orbit is not already filled, then it’s easier for the atoms to exchange or share those electrons and induce chemical reactions.
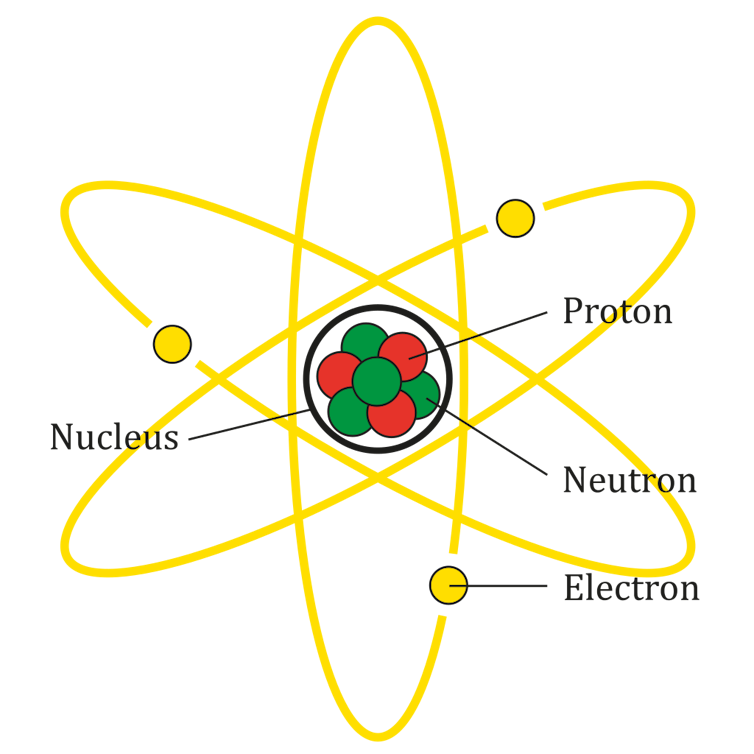
AG Caesar/Wikimedia Commons, CC BY-SA
One class of elements, the noble gases, hardly ever react with other elements. In noble gases, the electrons occupy completely filled orbits, and as a result the atoms greedily hold onto their electrons instead of sharing and undergoing a chemical reaction.
In the 1930s, scientists wondered whether protons and neutrons might also occupy orbits, like electrons. But nobody could show this conclusively. For more than a decade, the scientific community was unable to describe the nucleus in terms of individual protons and neutrons. Scientists used a more simplified picture, one that treated protons and neutrons as one single system, like a drop of water.
Magic numbers
In 1949, Goeppert Mayer and Jensen developed the so-called shell model of the nucleus.
Protons and neutrons occupy particular orbits, analogous to electrons, but they also have a property called spin – similar to a spinning top. Goeppert Mayer and Jensen found that when combining the two properties in their calculations, they were able to reproduce the experimental observations.
Through some experiments, they found that nuclei with certain magic numbers of neutrons or protons are unusually stable and hold onto their nucleons more than researchers previously expected, just like how noble gases hold onto their electrons.
The magic numbers known to scientists are 2, 8, 20, 28, 50, 82 and 126. They are the same for both protons and neutrons. When a nucleus has a magic number of protons or neutrons, then the particular orbit is filled, and the nucleus is not very reactive, similar to the noble gases.
For example, the element tin has a magic number of protons. Tin always has 50 protons, and its most common isotope has 70 neutrons. Isotopes are atoms of the same element that have a different number of neutrons.
There are nine other stable isotopes of tin that can exist – it’s the element with the largest number of stable isotopes. A stable isotope will never spontaneously change into a different element, which is what happens to radioactive isotopes.
Helium, with two protons and two neutrons, is the lightest “doubly magic” nucleus. Both its neutron count and its proton count are a magic number. The forces that hold the helium-4 nucleus together are so strong that it’s impossible to attach another proton or neutron. If you tried to add another proton or neutron, the resulting atom would fall apart instantaneously.
On the other hand, the heaviest stable nucleus in existence, lead-208, is also a doubly magic nucleus. It has magic numbers of 82 protons and 126 neutrons.
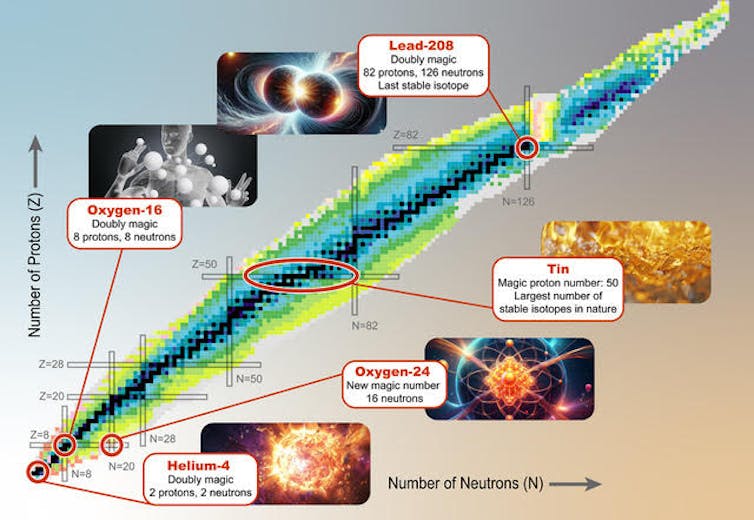
The Facility for Rare Isotope Beams
Examples of magic numbers and stable nuclei exist everywhere – but scientists couldn’t explain them without the introduction of the shell model.
Stable nuclei in nature
The shell structure in nuclei tells researchers about how elements are distributed across the Earth and throughout the universe.
One of the most abundant elements on our planet and in the human body is oxygen, in particular the isotope oxygen-16.
With eight protons and eight neutrons, oxygen-16 has an extremely stable nucleus. A nearby star produced the oxygen we find on Earth through nuclear reactions in its core sometime before the solar system was formed.
Since oxygen nuclei are doubly magic, these nuclei in the star did not interact very much with other nuclei. So more oxygen was left around to eventually act as an essential ingredient for life on Earth.
In her Nobel lecture, Maria Goeppert Mayer talked about the work she did with physicist Edward Teller. The two had attempted to describe how these elements formed in stars. In the 1930s, it was impossible for them to explain why certain elements and isotopes were more abundant in stars than others. She later found that the increased abundances corresponded to nuclei with something in common: They all had magic numbers of neutrons.
With the shell model and the explanation of magic numbers, the production of elements in stars was possible and was published in 1957.
Scientists today continue to use ideas from the nuclear shell model to explain new phenomena in nuclear science. A few accelerator facilities, such as the Facility for Rare Isotope Beams, where we work, aim to create more exotic nuclei to understand how their properties change compared with their stable counterparts.
At the Facility for Rare Isotope Beams, scientists produce new isotopes by accelerating stable isotopes to about half the speed of light and smashing them at a target. Out of the pieces, we select the rarest ones and study their properties.
Possibly the most profound modern discovery is the fact that the magic numbers change in exotic nuclei like the type we create here. So, 75 years after the original discovery, the race to discover the next magic number is still on.
Artemis Spyrou, Professor of Nuclear Physics, Michigan State University and Sean Liddick, Associate Professor of Chemistry, Michigan State University
This article is republished from The Conversation under a Creative Commons license. Read the original article.